Retroreflective Coating for Window Blinds
Reconciling view, solar control and visual comfort
Sign in and Register
Create an Account
Overview
Abstract
Solar shading devices are required to find a trade-off between conflicting requirements: Protection from excessive solar gains and glare, daylight provision, and visual contact with the environment. Conventional shading devices using absorbing or reflective materials typically trade the protection requirements against the others, and therefore occlude the glazing.
Retro-reflection of incident solar radiation towards the sky or the sun is an effective approach to the problem. The effect can be achieved in two dimensions, deflecting light to the incident elevation, but not azimuth angle, by prismatic structures forming either the profile or the surface structure of blinds. Achieving such shapes is elaborate due to the required precise processing of the louvers. Corner-cube structures applied as films to glazing have been demonstrated to achieve retroreflection in three dimensions (including the azimuth angle) of the invisible near-infrared portion of the solar spectrum, but only with the scope of moderating urban heat island effects caused by reflection from facades.
In this paper a novel approach is described and a first feasibility analysis is performed to achieve retro-reflection through a coating based on micro glass beads (spheres) for application on standard blind materials of thin/regular geometry.
The first prototypes of the coating turn out to be so effective that conventional spectrophotometric measurement (EN 14500) revealed to be useless, since it does not account for retro-reflection. This led to a completely new workflow including experimental measuring devices and modeling methods. The coating’s retro-reflection property was first modeled by raytracing and then by a data driven model obtained from gonio-photometric measurement. The coating models were applied to Venetian blinds, and compared to regular diffuse and mirror-like reflection in daylight simulation with Radiance.
The preliminary results show the great potential of the retro-reflective coating to design shading devices that can control solar gains and glare and motivate further engineering, developments and validation.
Authors
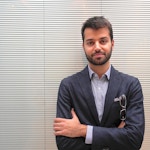
Luca Papaiz
Innovation Manager
Pellini SpA
Lpapaiz@pellini.net
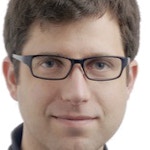
Lars Oliver Grobe
Lucerne University of Applied Sciences and Arts
larsoliver.grobe@hslu.ch
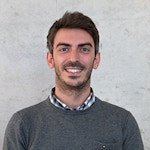
Giuseppe De Michele
EURAC Research
giuseppe.demichele@eurac.edu
Introduction
Shading devices that control solar gains to prevent overheating in summer, and excessive admission of daylight that potentially causes visual discomfort and glare, significantly affect electrical energy demand for heating
Access Restricted
Background
Standard Shading: Specular and Diffuse Reflectivity
Regardless of whether they are installed outside, inside or embedded into the glass building skin, solar shading devices work by absorbing or reflecting solar energy
Access Restricted
Method
Development of Retro-Reflective Coating
Previous attempts to design retro-reflective shading devices have been based on the development of advanced geometric profiles or structures with highly specular materials, and have led to
Access Restricted
Data
Model of the Coatings and Simulated Light Scattering
The BRDF of the virtual retro-reflective model is shown in Figure 10. The two images show the portion of energy reflected from the
Access Restricted
Explanation
Initial Performance Assessment
The analysis on the calculated models of the retro-reflective coating shows promising results in terms of visual performance. The luminance distribution over the façade is reduced when compared
Access Restricted
Conclusion and Future Work
The preliminary results of this research indicate the coating’s potential to reconcile aspects that usually are in direct contradiction when it comes to shading devices, namely: visual contact to the
Access Restricted
Acknowledgements
Figure 7a is based on an illustration contributed by Peter Apian-Bennewitz, pab advanced technologies ltd., Freiburg. This research was financially supported by the Swiss Innovation Agency Innosuisse and is part of the Swiss Competence Center for Energy Research SCCER FEEB&D. Part of the coating development was funded by the European Union’s Horizon 2020 research and Innovation programme under grant agreement N° 768766 – Energymatching.
Rights and Permissions
Breitenbach J., Lart S., Längle I., & Rosenfeld J.L.J. (2001). Optical and thermal performance of glazing with integral venetian blinds. Energy and Buildings 33, pp. 433-442. DOI:10.1016/S0378-7788(00)00102-X
De Michele G.,Loonen R., Saini H., Favoino F., Avesani S., Papaiz L., Gasparella A. (2018). Opportunities and Challenges for Performance Prediction of Dynamic Complex Fenestracion Systems (CFS). Journal of Facade Design & Engineering Vol.6 / Num.3 /pp. 101-115. DOI:10.7480/jfde.2018.3.2531.
EN 17037:2019. Daylight in buildings. Standard, European Committee for Standardization.
Grobe, L.O. (2018). Characterization and data-driven modeling of a retro-reflective coating in Radiance. Energy and Buildings 162, pp. 121-133. DOI: 10.1016/j.enbuild.2017.12.029
Köster H. (2004). Dynamic daylighting architecture: Basics, systems, projects. Birkhäuser, Basel.
Kuhn T.E. (2017). State of the art of advanced solar control devices for buildings. Solar Energy 154, pp. 112-133. DOI:10.1016/j.solener.2016.12.044
Mardaljevic, J., & Nabil, a. (2005). The Useful Daylight Illuminance Paradigm: A Replacement for Daylight Factors. Lux Europa, Berlin, 169–174.
Nicodemus, F. E. (1970). Reflectance nomenclature and directional reflectance and emissivity. Applied Optics, 9(6), 1474–1475.
Noback A., Grobe L.O., & Wittkopf S. (2016). Accordance of light scattering from design and de-facto variants of a daylight redirecting component. Buildings 6. DOI:10.3390/buildings6030030
Papaiz L., Pellini A. (2016) Double glazing system with retro-reflective properties. Patent: WO2017130110A1
Schregle R., Grobe L.O., & Wittkopf S. (2015). Progressive photon mapping for daylight redirecting components. Solar Energy 114, pp. 327-336. DOI:10.1016/j.solener.2015.01.041
Sun Y., Wu Y., Wilson R., & Lu S. (2016). Experimental measurement and numerical simulation of the thermal performance of a double glazing system with an interstitial Venetian blind. Building and Environment 103, pp. 111-122. DOI:10.1016/j.buildenv.2016.03.028
Tsangrassoulis A. (2016). Shading and Daylight Systems. In: Boemi S.N., Irulegi O., Santamouris M. (eds) Energy Performance of Buildings. Springer, Cham. pp. 437-466. DOI:10.1007/978-3-319-20831-2_21
Ward G., Kurt M., & Bonneel N. (2014). Reducing anisotropic BSDF measurement to common practice. In: Klein R.,
Rushmeier H. (eds) Eurographics workshop on material appearance modeling, Lyon (France), June. DOI:10.2312/mam.20141292
Ward, J, G. (1989). The RADIANCE Lighting Simulation and Rendering System. 21st Annual Conference on Computer Graphics and Interactive Techniques, 459–472. Retrieved from http://radsite.lbl.gov/radianc...
Ward, G. (2011). Computing and applying variable-resolution data for bidirectional scattering distribution functions, 1–19.
Wienold J., & Christoffersen J. (2006). Evaluation methods and development of a new glare prediction model for daylight environments with the use of CCD cameras. Energy and Buildings 38, pp. 743-757. DOI:10.1016/j.enbuild.2006.03.017
Wienold J., Iwata T., Sarey Khanie M., Erell E., Kaftan E., Rodriguez R., Yamin Garreton J., Tzempelikos T., Konstantzos I., Christoffersen J., Kuhn T.E., Pierson C.M., & Andersen M. (2019). Cross-validation and robustness of daylight glare metrics. Lighting Research & Technology, pp. . DOI: 10.1177/1477153519826003
Wienold, J., & Christoffersen, J. (2006). Evaluation methods and development of a new glare prediction model for daylight environments with the use of CCD cameras. Energy and Buildings, 38(7), 743–757. http://doi.org/10.1016/j.enbui...