Novel Active Facade Systems and Their Energy Performance in Commercial Buildings
Impact of Thermoelectric Materials on Heating and Cooling in Different Climates
Presented on October 12, 2022 at Facade Tectonics 2022 World Congress
Sign in and Register
Create an Account
Overview
Abstract
This article presents results of a research study that focuses on understanding energy performance of novel facade systems that integrate thermoelectric materials (TEs). TEs are active materials that can produce a temperature gradient when electricity is applied, exploiting the Peltier effect, or can generate a voltage when exposed to a temperature gradient, utilizing the Seebeck effect. In our previous research, we developed and tested working prototypes of these novel facades and investigated heating and cooling potential, as well as energy generation where temperature difference between the exterior and interior environments would be used to produce power from TEs, integrated into building skin. Previous research indicated that these intelligent systems are a promising new technology that can be used in facade assemblies for heating and cooling purposes, controlling buildings’ interior environment.
This research study considered a commercial office building and analyzed integration of these novel facade systems to provide localized heating and cooling. The main objective of the study was to compare energy performance of these novel facades to conventional HVAC systems. Our study was motivated in part by the fact that TEs are often considered to have inferior coefficient of performance compared to conventional building-scale HVAC systems due to the small size of TE modules. A baseline four-story office building with an area of 20,000 ft2 was developed in IDA ICE software program. Two different models were developed—one representing conventional HVAC system typically used in commercial buildings (VAV system), and one representing two systems, where the perimeter zone would integrate thermoelectric facades and the core would employ VAV system. Simulations were performed for fifteen climate zones in the U.S., investigating TE and VAV’s energy performance in various weather conditions. Therefore, 30 energy models were developed and simulated. Simulation results showed that energy consumption of a commercial office with integrated thermoelectric facades would be lower than conventional VAV system for all climate zones, with higher energy savings in colder climates because TEs act as heat pumps in heating mode, delivering higher efficiency.
Authors
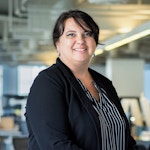
Ajla Aksamija
Professor and Chair
University of Utah
ajla.aksamija@utah.edu
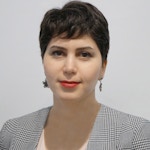
Mahsa Farid Mohajer
PhD Candidate
University of Massachusetts Amherst Department of Environmental Conservation
mahsafarid@umass.edu
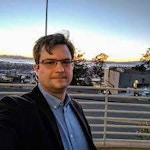
Zlatan Aksamija
Associate Professor
University of Utah
zlatan.aksamija@utah.edu
Keywords
1. Introduction and Previous Research
Active facade systems can be defined as building enclosures that react to the exterior environment, adapt to environmental changes, regulate their performance and functioning (by self-regulation or by users), integrate smart materials and components, and in some instances provide renewable energy for building’s operation. Active facade systems are an important aspect of advanced building technologies that can revolutionize our buildings, and significantly improve their performance.
This article reports on research focusing on integration of thermoelectric materials in facade assemblies to create intelligent building skins. Thermoelectric (TE) materials are active materials that can produce a temperature gradient when electricity is applied, exploiting the Peltier effect, or generate a voltage when exposed to a temperature gradient, utilizing the Seebeck effect. These types of materials can be used for heating and cooling, since heating and cooling modes can be switched by reversing the current direction.
Thermoelectric heating and cooling have several advantages over conventional counterparts. Physically, since TE modules have no moving parts, there are no mechanical components to degrade or generate noise. This greatly reduces maintenance costs, compared to other types of air conditioning systems (Boukai et al. 2008). Additionally, TE modules can convert electricity into thermal energy without the use of chlorofluorocarbons and can recover waste heat, converting it into electrical power. Thus, TE systems offer an opportunity to significantly reduce greenhouse gases emissions, and to generate clean energy supply without the use of toxic refrigerants (Upadhyaya, Khatami, and Aksamija 2015; Lee et al. 2012). The compact size of commercial TE modules, their light weight, reliability, lack of mechanical parts and elimination of the need for chlorofluorocarbons (CFCs) make them environmentally friendly and appealing. Previous research and development largely focused on thermoelectric modules (TEMs) that convert heat energy into electrical energy (Montecucco et al. 2012) and novel TE materials that offer higher energy efficiency through nanoscale engineering (Snyder and Toberer 2008). Given the high energy usage found in conventional HVAC systems, particularly on the heating side, coupled with potential leakage of greenhouse gasses on the cooling side, new heating and cooling sources are needed to reduce buildings’ overall carbon footprint. Moreover, integration of different building systems, particularly building envelope and HVAC, is essential for investigating intelligent, innovative facade assemblies. TEs are an example of a promising technology with potentially revolutionary architectural applications.
The overarching objective of this research is to investigate integration of TE materials into building skins to create active facade systems, which control the temperature difference between the exterior and interior environments to provide heating and cooling via heat pumping, or utilize that difference for energy generation via heat scavenging. Figure 1 shows the conceptual diagram and basic principles of operation. In our prior research investigating these novel facade systems, we employed experimental and quantitative research methods, including prototyping, testing, experiments, simulations and modeling. Initially, two prototypes were designed and constructed to test the viability of this concept, with different configurations. These prototypes were first tested in ambient room conditions to measure the heating and cooling outputs of TE materials. The temperatures that were measured during ambient testing ranged from 49°F (9°C) to 258°F (126°C) in both cooling and heating modes (Aksamija et al. 2018a). Then, an experimental study was employed to physically evaluate heating and cooling outputs, where a controlled thermal chamber was used to represent different exterior temperatures, and interior temperature was kept constant. The chamber was set to 90°F, 60°F, 30°F, and 0°F (32°C, 16°C, -1°C, and -18°C) to represent different exterior temperatures (winter, summer, and intermediate seasons). This method of testing represented typical exterior temperatures found in most climates, while allowing for temperature data to be collected in a controlled setting. The heating mode was tested under 60°F, 30°F, and 0°F (16°C, -1°C, and -18°C) conditions, while the cooling mode was tested under 90°F and 60°F (32°C and 16°C). Temperature measurements on the exterior surface of the prototype were recorded using a thermal camera. The results were promising and indicated that facade-integrated TE materials would be providing sufficient heating and cooling (Aksamija et al. 2018b, Aksamija et al. 2019).

Our previous research also investigated heating and cooling potentials of these novel systems for conditioning commercial office spaces (Aksamija et al. 2020). A typical commercial office space was used in the simulation study to investigate heating and cooling capabilities. In the simulation model, a single office space was modeled with an exterior wall consisting of a thermoelectric facade, and interior walls as adiabatic partition walls. Computational Fluid Dynamics (CFD) simulations were conducted for different scenarios, using SOLIDWORKS software program, varying the exterior environmental conditions and percentage of exterior wall coverage with thermoelectric components (5%, 10%, 15% and 20%). The exterior temperature was identical to the experimental study, considering 90°F, 60°F, 30°F, and 0°F (32°C, 16°C, -1°C, and -18°C). Simulations were conducted to calculate temperature distribution within the interior space for these different scenarios, and to determine heating and cooling outputs. Results indicated that 15% TE wall coverage was the most efficient option both for heating and cooling purposes within the simulated space.

Following the experimental and simulation studies, we developed design concepts for integrating TE materials into different facade types, which would create active systems. Figure 2 shows design strategies for various facade types. In all cases, the exterior heat sinks would be seamlessly integrated into exterior cladding since aesthetic considerations are important for any facade design. For example, aluminum fins can be used as exterior shading devices, but also as an external heat sink, connected to the TE materials with copper conducting system and interior heat sink (a radiant panel). Aluminum cladding in a rainscreen application can be used as an exterior heat sink. Lastly, in a curtain wall application, aluminum panel, acting as exterior heat sink, can be integrated into spandrel area. In all three cases, radiant panel is placed on the interior side, and would provide heating and cooling. Also, the facade-integrated TE system would be installed as a modular piece and would be insulated from the rest of the exterior wall. The modular nature of this system would be suitable for all building types, as well as retrofits of existing buildings.
Thermoelectrics are increasing in efficiency over time, following a similar arc as photovoltaics. Since they are heat pumps, their efficiency in thermal-to-electric conversion is always limited by the Carnot limit, which depends on the difference between hot and cold sides of the TE. The difference between actual and upper-bound (Carnot) efficiency depends on the figure-of-merit, zT. Currently, there is no known upper bound on zT but progress in increasing it beyond 1 has been challenging. As new materials with higher zT emerge, they will likely become commercialized and contribute to rising efficiency in future implementations of our idea.
2. Research Objectives and Methods
The goal of this research was to investigate energy performance of these novel, active facade systems. The objectives were:
- To investigate energy performance of TE facade systems in various climatic conditions (applied to commercial office buildings) and
- To compare energy usage of these active facade systems to conventional HVAC systems.
This study was conducted by modeling energy usage of a commercial office building, using IDA ICE software program. A baseline, rectangular four-story office building with an area of 20,000 ft2 (1,859 m2) and Window-to-Wall Ratio (WTW) of 40% was considered. Two different models were used for the study—one representing conventional HVAC system typically used in commercial buildings (VAV system), and one representing two systems, where the perimeter zone would integrate thermoelectric facades and the core would employ VAV system. For this scenario, the assumption was that the core area with the VAV system would be located 33 ft (10 m) from two short exterior walls and 16 ft (5 m) distant from the other two exterior walls. The exterior walls integrated TE facade systems for the perimeter zone (15% of exterior walls), which were modeled as radiant systems. Table 1 shows constant inputs that were used in the study for building systems and materials, operating schedules, lighting, and equipment loads.
Table 1: Constant inputs used for energy modeling.
Type | Inputs |
Exterior solid walls | Brick cavity wall with concrete back-up wall and insulation U-Value= 0.065 Btu/hft2F (0.372 W/m2K) |
Glazing system | Double insulated glazing unit, clear U-Value= 0.51 Btu/hft2F (2.9 W/m2K) SHGC=0.76 VT=0.81 |
Window-to-wall ratio | 40% |
Interior walls | Partitions U-Value= 0.3 Btu/hft2F (1.7 W/m2K) |
Ground floor | Concrete slab, insulated U-Value= 0.041 Btu/hft2F (0.23 W/m2K) |
Other floors | Concrete, 6 in (150 mm) U-Value= 0.42 Btu/hft2F (2.39 W/m2K) |
Roof | Concrete, insulated U-Value= 0.03 Btu/hft2F (0.17 W/m2K) |
Schedule | 8 am to 5 pm (weekdays) |
Number of occupants | 50 |
Equipment load | 3.75 kW |
Lighting load | 5 kW |
Simulations were performed for 15 climate zones in the U.S., investigating TE and VAV’s energy performance in various weather conditions. Table 2 shows considered climate zones. Therefore, 30 energy models were developed and simulated.
Table 2: Climate zones and representative cities used for the energy modeling.
Climate zone | City | Zone | Region | |
1 | 1A | Miami, FL | Very hot | Moist |
2 | 2A | Houston, TX | Hot | Moist |
3 | 2B | Phoenix, AZ | Hot | Dry |
4 | 3A | Memphis, TN | Warm | Moist |
5 | 3B | El Paso, TX | Warm | Dry |
6 | 3C | San Francisco, CA | Warm | Marine |
7 | 4A | Baltimore, MD | Mixed | Moist |
8 | 4B | Albuquerque, NM | Mixed | Dry |
9 | 4C | Salem, OR | Mixed | Marine |
10 | 5A | Chicago, IL | Cool | Moist |
11 | 5B | Boise, ID | Cool | Dry |
12 | 6A | Burlington, VT | Cold | Moist |
13 | 6B | Helena, MT | Cold | Dry |
14 | 7 | Duluth, MN | Very cold | |
15 | 8 | Fairbanks, AK | Subarctic |
The existing energy modeling software programs do not have the capability to model and simulate TE facade system, therefore, a representative system was used as a replacement for all energy models. To accurately model and capture TE system’s impacts on the energy performance, an electric radiator, with characteristics that most closely matched that of the TE system, was used. This included assigning a certain area to the radiator and calculating its rated input power based on the climate conditions. The assigned area for each electric radiator was set as 15 ft2 (1.4 m2). Given that the TE system’s performance and output depend on the temperature difference between the building internal and external environments, this was separately calculated for each climate zone and used for modeling the energy performance of TE system. Table 3 shows power rating calculations for various climate zones. This also implies that the efficiency of TE modules increases as the difference between the interior and exterior temperature increases. This data was used to assign input power ratings for the radiant system. As stated previously, TE facades were modeled as radiant systems, and conventional HVAC system considered single zone VAV system. The next section presents results of energy modeling in detail and discusses implications of these results.
Table 3: Temperature differences between the interior and exterior environments for considered climate zones, and associated power rating values for TE system.
Climate zone | Indoor temperature °F (°C) | Maximum outdoor temperature °F (°C) | Minimum outdoor temperature °F (°C) | Mean delta temperature °F (°C) | Power rating per module (W) | Total power rating (W) | |
1 | 1A | 70 (21) | 96 (36) | 41 (5) | 59 (15) | 80 | 2,160 |
2 | 2A | 70 (21) | 96 (36) | 33 (1) | 64 (18) | 78 | 2,106 |
3 | 2B | 70 (21) | 111 (44) | 36 (2) | 70 (21) | 75 | 2,025 |
4 | 3A | 70 (21) | 101 (38) | 11 (-12) | 77 (25) | 70 | 1,890 |
5 | 3B | 70 (21) | 102 (39) | 22 (-6) | 73 (23) | 75 | 2,025 |
6 | 3C | 70 (21) | 91 (33) | 36 (2) | 59 (15) | 80 | 2,160 |
7 | 4A | 70 (21) | 95 (35) | 6 (-14) | 77 (25) | 70 | 1,890 |
8 | 4B | 70 (21) | 101 (38) | 15 (-9) | 76 (24) | 70 | 1,890 |
9 | 4C | 70 (21) | 101 (38) | 13 (-11) | 77 (25) | 70 | 1,890 |
10 | 5A | 70 (21) | 95 (35) | -9 (-23) | 84 (29) | 63 | 1,701 |
11 | 5B | 70 (21) | 101 (38) | 3 (-16) | 81 (27) | 65 | 1,755 |
12 | 6A | 70 (21) | 99 (37) | -18 (-28) | 90 (33) | 55 | 1,485 |
13 | 6B | 70 (21) | 95 (35) | -12 (-24) | 86 (30) | 60 | 1,620 |
14 | 7 | 70 (21) | 91 (33) | -23 (-31) | 89 (32) | 57 | 1,539 |
15 | 8 | 70 (21) | 86 (30) | -37 (-38) | 94 (34) | 55 | 1,485 |
3. Results
The results included monthly and annual energy performance, as well as Energy Usage Intensity (EUI) for each analyzed climate zone. Monthly energy data combined various energy loads, including lighting, equipment
Access Restricted
4. Discussion and Conclusions
Results of this research indicated that TE materials are promising intelligent components that can be used in active facade assemblies for heating and cooling purposes, controlling buildings’ interior environment. This
Access Restricted
Rights and Permissions
Aksamija, Ajla, Zlatan Aksamija, Chris Counihan, Dylan Brown, D., and Meenakshi Upadhyaya. 2018a. “Thermoelectric Materials in Exterior Walls: Experimental Study on Using Smart Facades for Heating and Cooling in High-Performance Buildings”, Proceedings of the Facade World Congress 2018, Los Angeles, CA, March 12-13, pp. 171-180.
Aksamija, Ajla, Zlatan Aksamija, Chris Counihan, Dylan Brown, and Meenakshi Upadhyaya. 2018b. “Experimental Study on Integration of Thermoelectric Materials in Exterior Walls for Heating and Cooling in High-Performance Buildings”, Proceedings of the Building Enclosure Science and Technology (BEST) 5 Conference, National Institute of Buildings Sciences (NIBS), Philadelphia, PA, April 16-18.
Aksamija, Ajla, Zlatan Aksamija, Chris Counihan, Dylan Brown, and Meenakshi Upadhyaya. 2019. “Experimental Study of Operating Conditions and Integration of Thermoelectric Materials in Facade Systems”, Frontiers in Energy Research, Special Issue on New Materials and Design of the Building Enclosure, Volume 7, Article 6, DOI: 10.3389/fenrg.2019.00006.
Aksamija, Ajla, Zlatan Aksamija, Mahsa Farid Mohajer, Meenakshi Upadhyaya, and Guy Vignaeu. 2020. “Thermoelectric Facades: Simulation of Heating, Cooling and Energy Generation Potential for Novel Intelligent Facade Systems”, Proceedings of the Facade World Congress 2020, Los Angeles, CA, August 5-27.
Boukai, Akram I., Yuri Bunimovich, Jamil Tahir-Kheli, Jen Kan Yu, William A. Goddard, and James R. Heath. 2008. “Silicon Nanowires as Efficient Thermoelectric Materials.” Nature 451 (7175): 168–71. https://doi.org/10.1038/nature06458.
Lee, Eun Kyung, Liang Yin, Yongjin Lee, Jong Woon Lee, Sang Jin Lee, Junho Lee, Seung Nam Cha, et al. 2012. “Large Thermoelectric Figure-of-Merits from SiGe Nanowires by Simultaneously Measuring Electrical and Thermal Transport Properties.” Nano Letters 12 (6): 2918–23. https://doi.org/10.1021/nl300587u.
Montecucco, Andrea, James Buckle, and Andrew Knox. 2012. “Solution to the 1-D Unsteady Heat Conduction Equation with Internal Joule Heat Generation for Thermoelectric Devices.” Applied Thermal Engineering 35 (1): 177–84. https://doi.org/10.1016/j.applthermaleng.2011.10.026.
Snyder, G. Jeffrey, and Eric S. Toberer. 2008. “Complex Thermoelectric Materials.” Nature Materials 7 (2): 105–14. https://doi.org/10.1038/nmat2090.
Upadhyaya, Meenakshi, Seyedeh Nazanin Khatami, and Zlatan Aksamija. 2015. “Engineering Thermal Transport in SiGe-Based Nanostructures for Thermoelectric Applications.” Journal of Materials Research 30 (17): 2649–62. https://doi.org/10.1557/jmr.2015.202.