Modelling Perimeter Heating Demand
A Function of Occupant Thermal Comfort
Presented on October 13, 2022 at Facade Tectonics 2022 World Congress
Sign in and Register
Create an Account
Overview
Abstract
Thermal discomfort can occur in perimeter zones due to radiant heat loss from the occupant to a poorly insulated exterior wall. It is valuable for architects to be able to quantify this discomfort during early design stages when considering facade options. Current tools which facilitate this analysis ignore the perimeter heating systems which would normally compensate for radiant heat loss. Therefore, it is difficult to argue that one facade creates more comfortable conditions than another. This paper proposes an alternative approach for bringing thermal comfort and energy quantities into early stage design discussions. As part of the approach, the exact perimeter heating energy demand required to deliver thermally comfortable conditions is quantified. A Python model for producing this quantity is demonstrated using the example of a typical Toronto multi-unit residential building. The results showed that a unit with a High Performance facade would require 60% less perimeter heating energy than a unit with a Low Performance facade. Peak heating demand from a radiant ceiling panel ranged from 350 W to 1460 W for the highest and lowest performing facades respectively. Overall, the model is shown to be effective at assessing the performance of facades, and capable of building an argument for higher performance facades. However, future improvements should address radiant asymmetry and transmitted solar radiation.
Authors
Jonathan Graham
Sustainability Analyst
KPMB Architects
jgraham@kpmbarchitects.com
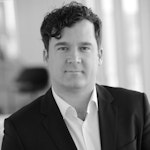
Geoffrey Turnbull, OAA, MRAIC, LEED AP, CPHD
Director of Innovation
KPMB Architects
gturnbull@kpmbarchitects.com
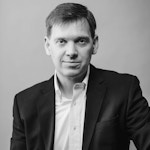
David Constable OAA, LEED AP, CPHD
Principal
KPMB Architects
dconstable@kpmbarchitects.com
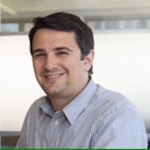
Mike Reimer P.Eng, LEED AP
Principal
First Light Energy Solutions
mike@firstlightenergy.ca
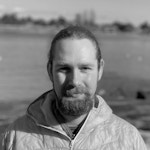
Joshua Vanwyck, CPHD
CEO
JMV Consulting
josh@jmvconsulting.ca
Keywords
Introduction and Background
As the focus of high performance building design expands from a focus on mechanical systems to include a focus on envelope design, it is useful for architectural designers to be able to understand the performance characteristics of various facade configurations at an early stage of design. Indeed, emerging regulatory requirements for high performance buildings often require guarantees of overall building performance, thermal energy demands, etc. at early stages of design (e.g. conclusion of schematic design phase.) Moreover, it is increasingly important for architects to be able to understand the impact of facade design decisions on occupant thermal comfort, quality of daylighting, etc. at an early stage, when it is easiest to align the building massing, plan, section, envelope strategies, etc. to produce optimal outcomes.
Occupant thermal comfort is influenced by the indoor air temperature, mean radiant temperature, solar radiation, air velocity and humidity. Accurate modelling of these parameters requires knowledge of the mechanical systems used, internal heat gains, and the thermal conductance and capacitance of surrounding materials (Berardi and Soudian 2019). This information is typically unavailable during schematic design phase, which signals the need for simplified thermal comfort models.
A handful of tools have the ability to evaluate indoor thermal comfort using the limited information available during early stages of design (Hoyt 2016, Menchaca-Brandan, et al. 2017). These tools require basic information about the indoor and outdoor environmental conditions, the glazing geometry, and the target window U-value. These inputs are easily obtainable during schematic design. However, most of these tools assume the absence of perimeter heating. This muddles potential arguments that higher performance facades result in better thermal comfort, because a properly designed perimeter heating system should inevitably compensate for facade-induced discomforts (Berglund, Yoshino and Kuno 1997).
Despite this logical gap in efforts to link facade performance to indoor thermal comfort during early stages of design, it is clear that a lower performance facade should require more heating energy to maintain an acceptable level of comfort. The exact quantity of this energy, if it could be isolated, would be descriptive of both facade performance and indoor thermal comfort.
This paper aims to demonstrate how thermal comfort and energy quantities can be brought into discussions about facade performance during early stages of design. This is achieved through a novel approach wherein the perimeter heating energy demand is treated as the energy required to deliver thermally comfortable conditions. A purpose-built Python model is introduced and tested using an archetypal Toronto residential suite as an example. The results of heating energy demand, peak heating demand and peak radiant panel temperature are reported. Lastly, intricacies and limitations of the modelling approach are discussed.
Method
Experiment Overview
A model was developed to quantify the heating energy required to maintain thermal comfort in the perimeter zone of a residential suite in Toronto, Ontario. The modelled space
Access Restricted
Results
The view factors between the occupant and each of the surfaces is shown in Table 2. The largest view factor was to the ceiling, floor and interior walls (“other interior
Access Restricted
Discussion
The experiment demonstrated the expected relationships between facade thermal performance and the heating energy delivered to the suite. Facades with low overall U-values and low WWRs required significantly less energy
Access Restricted
Conclusion
As high performance building design increasingly places value on the role of envelopes, it is useful for architects to be able to understand the performance implications of facade configurations at
Access Restricted
Rights and Permissions
Arens, Edward, Tyler Hoyt, Xin Zhou, Li Huang, Hui Zhang, and Stefano Schiavon. 2015. "Modeling the comfort effects of short-wave solar radiation indoors." Building and Environment 3-9.
ASHRAE. 2020a. ANSI/ASHRAE Standard 55-2020: Thermal Environmental Conditions for Human Occupancy. ASHRAE.
ASHRAE. 2020b. ASHRAE Handbook—HVAC Systems and Equipment. ASHRAE.
ASHRAE. 2021. ASHRAE Handbook—Fundamentals.
ASHRAE.
Berardi, Umberto, and Shahrzad Soudian. 2019. "Experimental investigation of latent heat thermal energy storage using PCMs with different melting temperatures for building retrofit." Energy & Buildings 185: 180-195.
Berglund, Larry G., Hiroshi Yoshino, and Satoru Kuno. 1997. "Comfort near cold perimeter walls through radiant ceiling panel and other compensation methods." IAQ 1997 -- Design, Construction, and Operation of Healthy Buildings: Solutions to Global and Regional Concerns. ASHRAE. 210-216.
Curcija, Charlie, Simon Vidanovic, Robert Hart, Jacob Jonsson, and Robin Mitchell. 2018. Window Technical Documentation. Windows and Envelope Materials Group, Berkeley: Lawrence Berkeley National Laboratory.
Environment Climate Change Canada. 2020. CWEEDS/CWEC/TDY Files – 2020 Release. Engineering Climate Services Unit, Meteorological Service of Canada, Toronto: Environment Climate Change Canada.
Holmgren, William F., Clifford W. Hansen, and Mark A. Mikofski. 2018. "pvlib python: a python package for modeling solar energy systems." Journal of Open Source Software 3 (29): 884.
Hoyt, Tyler. 2016. CBE MRT tool: Online user guide. Accessed September 28, 2021. https://github.com/CenterForTheBuiltEnvironment/mrt.
Huizenga, Charlie, Hui Zhang, Pieter Mattelaer, Tiefeng Yu, Edward Arens, and Peter Lyons. 2005. Window Performance for Human Thermal Comfort - Final Report. Presentation, Berkeley: Center for the Built Environment.
Menchaca-Brandan, M. Alejandra, Vera Baranova, Lynn Petermann, Stephanie Koltun, and Christopher Mackey. 2017. "Glazing and Winter Comfort Part 1: An Accessible Web Tool for Early Design Decision-Making." Proceedings of the 15th IBPSA Conference. San Francisco: IBPSA. 2449-2456.
Ozkan, Aylin, Ted Kesik, A. Zerrin Yilmaz, and William O’Brien. 2018. "Development and visualization of time-based building energy performance." Building Research & Innovation.
Sadeghipour Roudsari, Mostapha, and Michelle Pak. 2013. "Ladybug: a parametric environmental plugin for Grasshopper to help designers create an environmentally-conscious design." Proceedings of the 13th International IBPSA Conference. Lyon (France): IBPSA. 25-30.
Tartarini, Federico, and Stefano Schiavon. 2020. "pythermalcomfort: A Python package for thermal comfort research." SoftwareX 12: 100578.
Tredre, Barbara E. 1964. "Assessment of mean radiant temperature in indoor environments." British Journal of Industrial Medicine 22: 58-66.
Zani, Andrea, Henry D Richardson, Alberto Tono, Stefano Schiavon, and Edward Arens. 2019. "A simulation-based design analysis for the assessment of indoor comfort under the effect of solar radiation." Proceedings of SimAUD 2019. Atlanta: Society for Modeling & Simulation International (SCS).