Addressing Embodied Carbon
Managing Insulating Glass Service Life
Presented on October 12, 2022 at Facade Tectonics 2022 World Congress
Sign in and Register
Create an Account
Overview
Abstract
Reducing greenhouse gas emissions from the building sector is critical to limiting global temperature rise to less than 1.5⁰C. Construction and building operations account for 39% of all carbon emissions. Reducing both embodied (11%) and operational (28%) carbon emissions is critical. Extending the operational life of buildings and their components is a crucial, but often overlooked, lever in carbon reduction.
The advent of the insulating glass unit (IGU) has brought about significant improvements in operational energy efficiency of buildings. However, from an embodied carbon perspective, we have transformed a recyclable material that could last 100’s of years (single pane glass) into a system with a much shorter lifespan of around 20 to 40 years with more challenges associated with recycling.
The service life of an IGU is determined primarily by how long it can prevent moisture accumulation between lites. This is related to the type, quality, and quantity of materials used in the perimeter edge seal, the appropriateness of the IGU edge seal design for the façade application, the quality of the manufacturing, and the quality of the installation. The current practice is to use insulating glass seal weathering tests with defined conditions and accompanying performance specifications, to assess the serviceability of IGU designs, and certification programs to control the on-going manufacturing quality.
Accelerated weathering tests, such as ASTM E2188 and EN1279-2, typically deliver a defined range of loads on the IGU edge seal, to qualify edge seal performance. However, actual IGU constructions, applications, and environmental conditions (e.g., change in elevation, cavity temperature, or pressure) in real installations can result in much higher edge forces than simulated in accelerated weathering tests. While the stress in the edge seal is typically analyzed in structural glazing applications for capacity at the design wind load, the impact of environment-induced strain on the edge seal is not routinely evaluated. Control of IGU edge seal strain is key to managing service life, since stiffness of the edge sealant and spacer controls the strain on the primary sealant, which in turn controls the moisture vapor transmission. This paper provides a roadmap for assessing edge seal strain and for adjusting edge seal design to accommodate environmental loads, in order to help improve IGU service life and reduce embodied carbon by extending the replacement cycle.
Authors
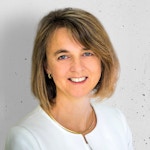
Helen Sanders, PhD
General Manager
Technoform North America
helen.sanders@Technoform.com
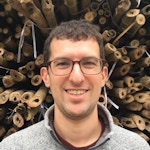
Adam Nizich
Senior Consulting Engineer
Simpson Gumpertz & Heger
ajnizich@sgh.com
Keywords
Introduction
The acute need to drive down greenhouse gas (GHG) emissions to avert the adverse effects of climate change requires us to evaluate and reduce the embodied carbon associated with the materials used to construct buildings. According to U.N. Environment’s Global Status Report 2017 [1], buildings and construction account for 39% of the carbon emissions worldwide, split approximately 70%/30% between building operations (operational carbon), and building materials and construction (embodied carbon). And yet, we are in a construction boom. The report predicted 230 billion square meters of buildings will be added worldwide by 2060, doubling the current building stock.
Careful low embodied carbon material and design choices are important, but they should be done within the context of a service life consideration. A low embodied carbon material choice which lasts only 10 years compared to a higher embodied carbon choice that lasts 30 years with a similar operational performance is likely not a good trade off. Similarly, a component that provides incrementally better building thermal performance (operational carbon) but reduces the service life of the component may also not be a good trade-off. The metric of embodied carbon per year of service life should be considered when comparing building components.
Studies have shown that the building envelope contributes to approximately 15% of the building’s embodied carbon, driven by carbon intensive materials such as insulation, aluminum, and glass [2]. This paper focuses on the impacts of the transparent elements of the façade. The flat glass manufacturing process is energy intensive, dominated by the burning of natural gas. Currently, no electrification or non-fossil fuel energy source has currently been shown to be feasible, although initial experiments with hydrogen fuel have begun [3]. The embodied carbon of insulating glass is dominated by that of the flat glass, which contributes approximately 75% of a dual pane’s GHG emissions, according to a processed glass Environmental Product Declaration by Vitro Architectural Glass [4].
In moving from single pane glass to insulating glass, we have significantly improved the operational carbon performance of buildings, but in exchange we have turned a material that could last many hundreds of years into one that lasts only 20 to 40 years (figure 1). Typically, there is no recycling stream at the end of life and disposal is in a landfill. Markets for reusing architectural glass in new windows are currently limited. Low-e coatings on glass, interlayers, and sealants used for bonding make recycling a challenge. Generally, if glass is diverted from a landfill, it is downcycled into insulation and road surfacing material. Therefore, one of the most important considerations in managing embodied carbon related to insulating glass specification is designing for longevity.
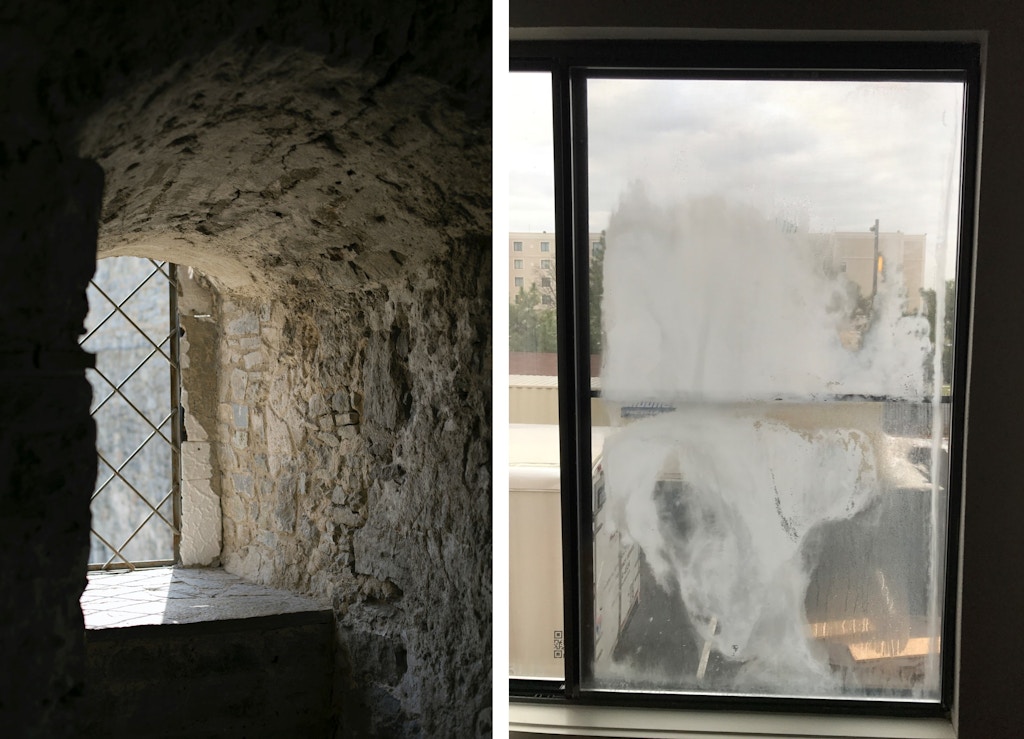
Background
The service life of an insulating glass unit (IGU) is primarily determined by how long it can prevent moisture accumulation between the lites of glass. There is always a finite
Access Restricted
Method
The following steps outline a method for assessing the edge seal forces for IGUs in project applications and identifying whether action is needed to adjust the IGU configuration, size, or
Access Restricted
Optimizing IGU Service Life Further
In addition, there are several other key factors that need to be considered which impact service life:
1. Design margin
The type, quality, and quantity of materials in the edge
Access Restricted
Conclusion
Extending the useful service life of façade components is critical to reducing both embodied and operational carbon emissions. The design of the IGU edge seal is critical to optimizing its
Access Restricted
Rights and Permissions
[1] UN Environment Programme, Global Status Report 2017, prepared by the International Energy Agency for the Global Alliance for Buildings and Construction.
[2] Paula Melton. The urgency of embodied carbon and what you can do about it. Building Green, Volume 27, Issue 9, September 10, 2018. https://www.buildinggreen.com/...
[3] NSG/Pilkington Plant in UK Manufactures float glass using hydrogen power in world-first trial, Glass Magazine, August 31, 2021, https://www.glassmagazine.com/...
[4] Vitro Architectural Glass Processed Glass Products Environmental Product Declaration, July 2017.
[5] ASTM C1249 -18 Standard Guide for Secondary Seal for Sealed Insulating Glass Units for Structural Sealant Glazing Applications
[6] A.W. Lingnell and J.L. Spetz. Field Correlation of Performance of Insulating Glass Units in Buildings – A 25-year study. ASHRAE, 2007. https://web.ornl.gov/sci/buildings/conf-archive/2007%20B10%20papers/066_Lingnell.pdf
[7] A. Nizich, H. Sanders, K. Burkhart. A Strain-Normalized Serviceability Limit State for the Secondary Seal of Insulating Glass Units with Rigid Box Spacers. Accepted for publication by ASTM International, publication pending.
[8] DIN 18008-1:2010-12. Glass in Building - Design and Construction Rules - Part 1: Terms and General Bases, English Translation. Berlin: Deutsches Institut fur Normung E.V., 2010.
[9] J. Griffith, S. Moore, I. Chaires, A. Goehring, and F. Weber. Climate Load Design Parameters for the United States. Glass Performance Days, Finland, 2019.
[10] EN 16612:2019. Glass in Building-Determination of the Lateral Load Resistance of Glass Panes by Calculation, EVS-EN 16612. Tallinn: Estonian Centre for Standardization and Accreditation, 2019.
[11] North American Glazing Guidelines for Sealed Insulating Glass Unites for Commercial and Residential Use, TM3000-90(16) published by IGMA.